Our solar system consists of the Sun at its center, surrounded by eight primary planets that orbit in nearly circular paths. These planets are arranged in a specific order from the closest to the farthest from the Sun: Mercury, Venus, Earth, Mars, Jupiter, Saturn, Uranus, and Neptune. Each planet maintains its path, adhering to orbital mechanics discovered by early astronomers like Galileo and Kepler, which help predict their movements and relative positions at any given time. The proximity of these planets to each other and Earth varies depending on their position in their orbits, leading to fascinating phenomena such as alignments and oppositions.
Understanding Planetary Distances
How Distance is Measured in Space
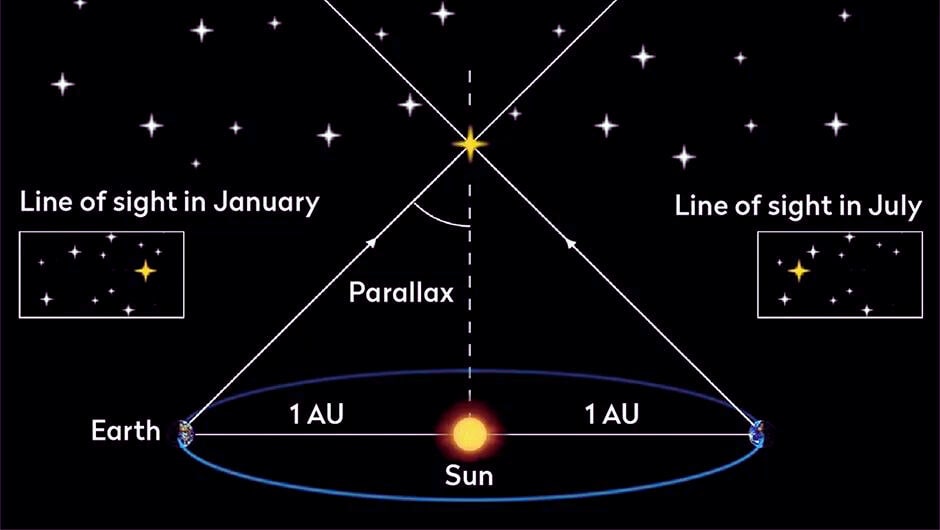
Distance measurement in space uses astronomical units such as the light year, which is essential due to the immense scales involved. A light year is the distance light travels in one year, approximately 5.88 trillion miles (9.46 trillion kilometers). This unit is particularly useful because it communicates the vast distances between stars and galaxies in terms that relate to the speed of light, making it easier to grasp these enormous scales.
Another common unit is the parsec, which is about 3.26 lightyears. Parsecs are often used in professional astronomy to measure distances between celestial objects outside our solar system. The choice of light years or parsecs depends on the context and the specific distances being measured.
Distances within our solar system, however, are typically measured in astronomical units (AU). One AU is the average distance from the Earth to the Sun, about 93 million miles (150 million kilometers). This unit simplifies the description and calculation of orbital elements within our solar system.
Measuring these vast distances involves observing the time it takes for light to travel from celestial bodies to Earth, often involving complex calculations of celestial mechanics and the effects of relativity in cases of extremely large distances.
Variables Influencing Proximity
The proximity of a planet to its star, crucial for climate and environmental conditions, is largely influenced by its orbital pattern and eccentricity. Orbital eccentricity measures how much an orbit deviates from a perfect circle, where a value of zero represents a circular orbit and higher values indicate more elliptical orbits.
- Eccentricity: A planet with high orbital eccentricity will have a significant variation in its distance from the star throughout its orbit. This affects not only the orbital velocity but also the closest and farthest points in its orbit relative to the star, impacting the planet’s climate and seasonal extremes.
- Kepler’s Laws: These laws describe how celestial bodies orbit. According to Kepler, the shape of the orbit (defined by eccentricity) dictates how a planet moves faster when closer to its star and slower when farther away, which can lead to varying degrees of solar radiation received over a year.
- Milankovitch Cycles: These cycles illustrate how shifts in orbit and eccentricity over thousands of years affect Earth’s climate. The precession of the equinoxes combined with eccentricity determines at which point in the orbit Earth is closest to the sun, thus influencing the timing and intensity of seasons.
Understanding these dynamics is vital for studying not just Earth’s climate history but also the habitability and environmental conditions of other planets within and beyond our solar system.
The Contenders
Venus
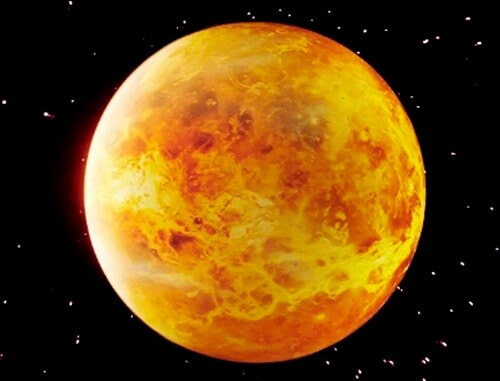
Venus, often referred to as Earth’s “twin” due to its similar size and proximity, holds several distinctive features and a unique orbit:
- Orbit and Distance: Venus orbits the Sun at a mean distance of approximately 108 million km (about 67 million miles), which is roughly 0.7 times the distance of Earth from the Sun. It has an extremely low orbital eccentricity, making its orbit almost circular.
- Rotation: Uniquely among the Solar System’s planets, Venus spins on its axis in a clockwise direction, opposite to most planets. This is known as retrograde rotation.
- Physical Characteristics: Venus is the hottest planet in our solar system, with surface temperatures sufficient to melt lead, mainly due to its thick atmosphere filled with carbon dioxide and clouds of sulfuric acid.
- Sidereal Day and Year: Interestingly, a day on Venus (the period for one complete rotation on its axis) is longer than its year (the period to complete one orbit around the Sun). It takes about 224.7 Earth days to complete an orbit, but a sidereal day on Venus lasts 243 Earth days.
These features make Venus a planet of extremes and contrasts, particularly intriguing despite its hostile conditions.
Mars
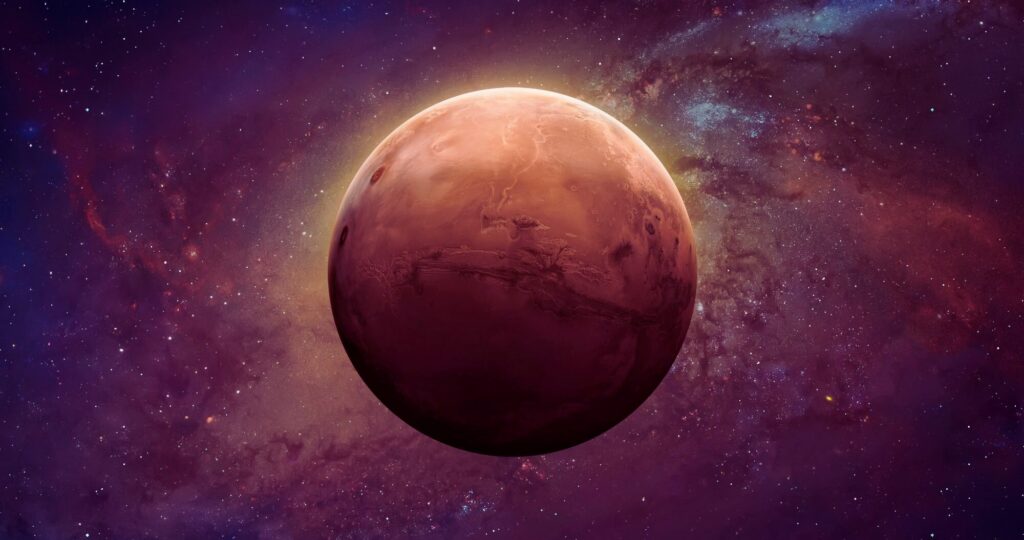
Mars, known as the Red Planet, has unique orbital and physical characteristics that distinguish it within our solar system:
- Orbit: Mars orbits the Sun at an average distance of about 228 million km (142 million miles) or 1.52 AU from the Sun. This orbit places Mars as the fourth planet from the Sun and involves a somewhat elliptical path, which means that its distance from the Sun varies significantly during a Martian year.
- Physical Characteristics: Mars has a diameter of approximately 6,779 km (4,212 mi), making it the second smallest planet in the Solar System after Mercury. Its size and mass influence its gravity, which is only about 38% that of Earth’s, affecting the atmospheric retention and surface conditions.
- Geological Features: The Martian surface is well known for having the largest volcano in the Solar System, Olympus Mons, and the longest, deepest canyon, Valles Marineris. These features underscore Mars’s geologically active past and provide significant insight into its geological evolution.
- Observation from Earth: Due to its external orbit relative to Earth’s, Mars is often very visible in the night sky and its appearance can be quite pronounced when it is opposite the Sun, at which point it is also closest to Earth.
These attributes make Mars a primary target for scientific study and exploration, particularly in the ongoing search for past life and potential for human colonization.
A Closer Look at the Measurements
Average Distances
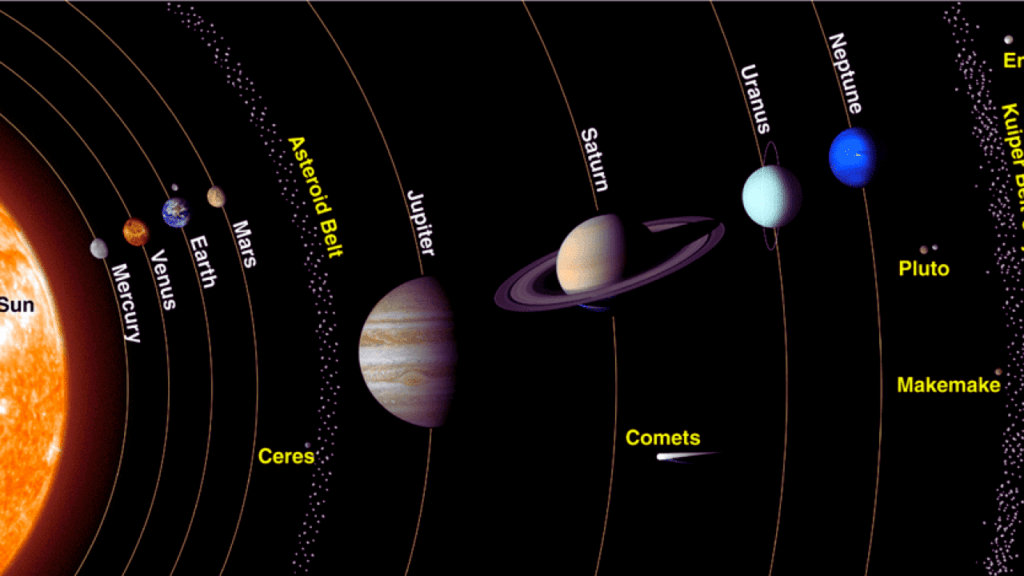
To statistically compare average distances between different sets of data points, various methods can be employed depending on the nature of the data and the specific requirements of the analysis. Here are some approaches based on the provided sources:
- Statistical Significance Testing: When determining if the average distance between vectors in different groups significantly differs, statistical tests like t-tests or ANOVA can be used. These tests help assess whether observed differences in means (average distances in this context) are likely due to random chance or actual differences in data distributions.
- Comparative Methods: For comparing distances between two objects or the areas of multiple objects, specialized methods like those described by Howson can be useful. These techniques may involve calculations that compare not only distances but also spatial relationships and area coverage, which can be crucial in fields like geographic information systems (GIS) and spatial analysis.
- Using Statistical Distance: Statistical distance measures, such as the Mahalanobis distance, Kullback-Leibler divergence, or the Earth Mover’s Distance, quantify the difference between probability distributions. These measures are valuable in scenarios where you need to compare how one statistical population differs from another in a probabilistic or information-theoretical context.
- Visual and Descriptive Methods: Utilizing visual mapping techniques alongside descriptive statistics can provide a more intuitive understanding of distance measurements. This approach is especially useful in health services research and environmental studies, where spatial distributions play a significant role.
These methods enable a robust analysis of distances, providing insights into the relationships and differences between data sets in various scientific and analytical contexts.
Closest Approach
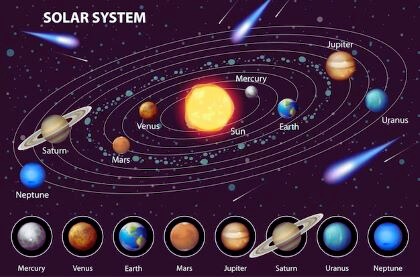
The closest approach of planets to Earth varies greatly depending on the planetary orbits and alignment with Earth and the Sun. Here’s a look at when some of the planets typically come closest to Earth:
- Mars: The Red Planet comes closest to Earth about every 26 months, an event closely related to Mars Opposition and Mars Retrograde. During the closest approach, Mars and the sun are on directly opposite sides of Earth, allowing Mars and Earth to be at their nearest. The last closest approach recorded was on May 31, with Mars being 75.28 million kilometers away from Earth.
- Jupiter: Jupiter’s closest approach to Earth happens approximately every 13 months, usually at or near its opposition when Earth is between Jupiter and the Sun. During the last significant event, Jupiter was notably close, marking its nearest distance in 59 years on September 26, 2022.
- Neptune: Although further details about Neptune’s specific close approaches are less discussed, it was noted to have its closest approach on September 11 in a recent year, with the next close event expected roughly around the same time the following year.
These close approaches provide exceptional opportunities for observation and study, offering astronomers clearer views and more detailed scientific data.
The Surprising Answer
The True Proximity

Despite commonly held beliefs, recent studies and calculations show that Mercury, not Venus, is the closest planet to Earth on average. Mercury orbits the Sun more closely than any other planet, which influences its average distance to Earth and other planets in the solar system. This proximity results from Mercury’s orbit, allowing it to be nearer to Earth more frequently than Venus, despite the latter sometimes being closer at specific points in their respective orbits.
Why Mercury is the Closest
Orbital Dynamics
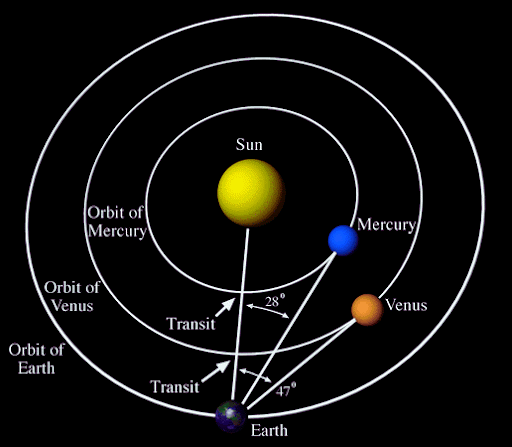
Mercury’s proximity to Earth can be attributed to its unique orbital characteristics. Firstly, Mercury is the closest planet to the Sun and orbits faster due to its proximity, completing an orbit in just about 88 Earth days. This rapid orbital speed means that Mercury frequently finds itself on the same side of the Sun as Earth, allowing it to be relatively closer compared to other planets that orbit more slowly.
Moreover, Mercury reaches its point of closest approach to the Sun, known as perihelion, during which it moves the fastest in its orbit. This dynamic, combined with the planet’s small orbital circumference, significantly reduces the average distance between Mercury and Earth compared to other planets, making it the closest on average despite the eccentricity of its orbit.
Comparative Orbital Speeds
Mercury is the fastest orbiting planet in our solar system, traveling around the Sun at an impressive speed of approximately 47.87 km/s. This is significantly faster compared to its neighboring planets, Venus and Mars. Venus orbits the Sun at about 35.02 km/s, making it the second fastest, while Mars trails behind with an orbital speed of about 24.07 km/s. This difference in orbital speeds is largely due to the varying distances from the Sun, which influences gravitational pull and resultant orbital velocities. Mercury, being closest to the Sun, moves faster to balance the strong gravitational pull it experiences.
The Role of Technology in Understanding
Advances in Observational Technology
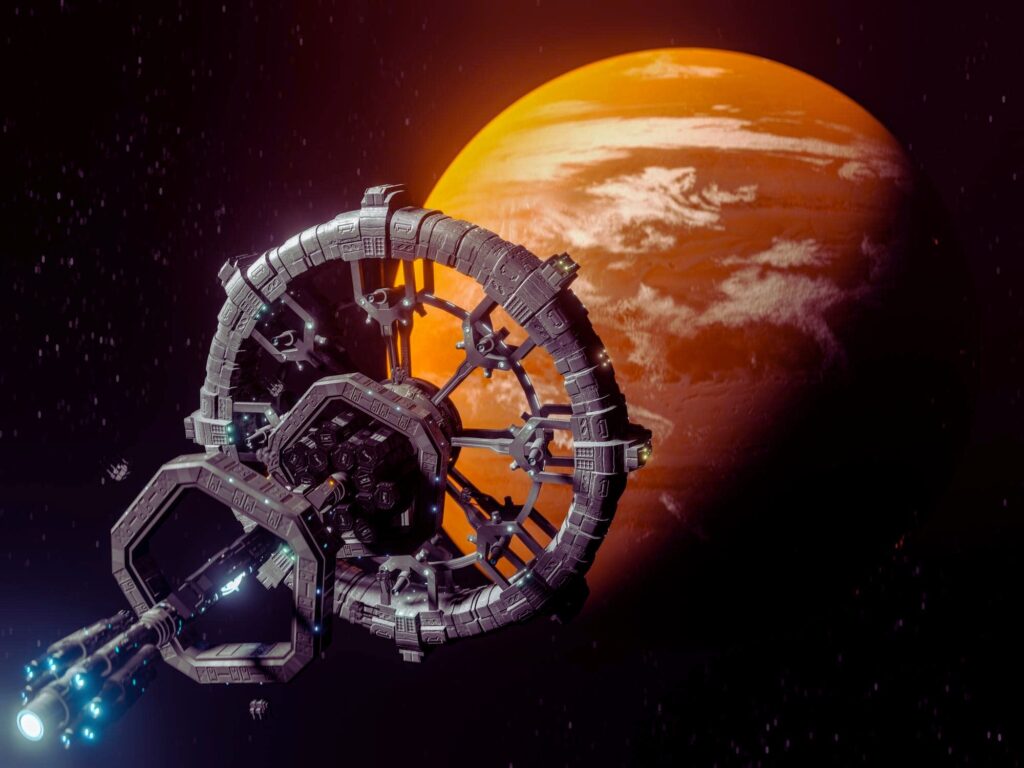
The past few centuries have seen significant technological advances that have profoundly expanded our understanding of the universe. Key developments include:
- Telescopes: The evolution of optical telescopes has dramatically enhanced our ability to observe distant celestial bodies and phenomena. Modern telescopes are equipped with high-resolution capabilities and can operate in various electromagnetic spectra, not just visible light.
- Automation and Computers: The automation of telescopes and the integration of computer technologies have revolutionized how astronomical data is collected. Observatories now use sophisticated software to manage and analyze vast amounts of data, significantly speeding up research and discovery.
- Detector Technology: Advances in quantum physics have led to the development of charged-coupled devices (CCDs) which are crucial for capturing images of the universe at unprecedented resolutions. These devices are sensitive to the minute quantities of light from distant stars and galaxies, allowing for more detailed observations than ever before.
These advancements have not only deepened our understanding of the cosmos but also increased our capacity to explore questions about the origins of the universe, the nature of dark matter, and the possibility of life beyond Earth.
Future Technologies
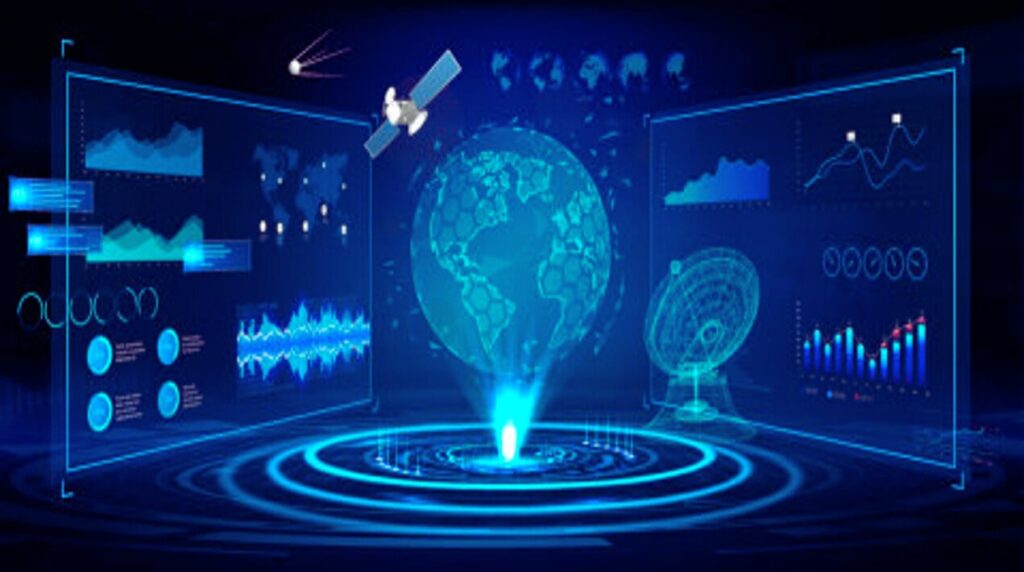
The future of technology in 2024 and beyond is expected to bring significant changes across various sectors. Here are some key trends to watch:
- Generative AI: This technology is moving from experimental to practical, widespread use in enterprise settings, driving new efficiencies and capabilities in automation and data analysis.
- Sustainable AI Infrastructure: There will be an increased focus on creating energy-efficient AI systems that are more environmentally friendly.
- Cyber Resilience: Enhancements in cybersecurity are expected as organizations prioritize protecting digital assets against increasingly sophisticated threats.
- AI-Driven Search and Apps: Improvements in generative AI search functionalities and the emergence of AI application stores will customize user experiences to unprecedented levels.
- Blockchain: There will be greater adoption of blockchain technologies, potentially reshaping financial transactions and data storage systems.
These developments suggest a future where technology not only enhances operational efficiencies but also contributes to sustainability and security.
Implications of Proximity
Practical Implications
Proximity to objects in space significantly influences both missions and scientific research in various ways:
- Efficient Material Analysis: Proximity allows missions to gather detailed material samples from celestial bodies such as asteroids. A study by JAXA highlighted that material blasted from ancient asteroids could be crucial for understanding the early solar system.
- Enhanced Planetary Defense: Proximity is key in planetary defense strategies. NASA’s DART mission exemplifies how nearby operations allow for the effective alteration of an asteroid’s trajectory to protect Earth.
- Detailed Impact Analysis: Understanding how impacts affect planetary bodies depends on observing these events up close. Research discussing impacts on planetary bodies shows that proximity provides critical data necessary for modeling and predicting future collisions.
Proximity in space missions allows for more precise measurements, effective intervention, and detailed analysis of celestial phenomena, ultimately leading to better scientific understanding and safer space exploration practices.
Philosophical and Educational Implications
Understanding our solar system has profound philosophical and educational implications, shaping our perception of the universe and our place within it:
- Conceptual Shifts in Learning: Research indicates that students’ ideas about the solar system can evolve significantly with targeted educational interventions, suggesting that teaching methods can greatly influence understanding of complex astronomical concepts.
- Philosophical Relevance: Kant’s discussion on space and time highlights the philosophical challenge in conceptualizing the universe. His ideas suggest that our understanding of space not only shapes scientific thought but also fundamental philosophical ideas about existence and the nature of reality.
- Integration of Philosophy in Science Education: Incorporating philosophical perspectives into science education, such as through courses on the Philosophy and Science of Space Exploration, can help address and reduce distrust in science. This approach fosters a deeper understanding and critical thinking about space exploration and its broader implications.
These insights reveal that learning about our solar system is not only about acquiring scientific knowledge but also involves enriching our philosophical understanding and challenging our perceptions of the cosmos.
Conclusion
While Venus and Mars often steal the spotlight when it comes to neighboring planets, it’s Mercury that, on average, spins closest to our Earth. This insight not only tweaks our galactic address book but also highlights the dynamic and ever-changing nature of our solar system.
Read also: What is Meta AI?
FAQs
Q. Why is Mercury often closer to Earth than Venus, despite being further from the Sun?
Mercury’s faster orbit means it crosses Earth’s path more frequently, making it often closer than the other planets.
Q. How do scientists measure the distances between planets?
Scientists use astronomical units and light years, along with radar and optical measurements, to calculate distances.
Q. What impact does the proximity of a planet have on space missions?
Closer proximity can make missions more feasible in terms of travel time and fuel requirements.
Q. Could new technologies change our understanding of which planet is closest to Earth?
Absolutely! As measurement technologies improve, our understanding of distances and planetary dynamics can be refined further.
Q. Why is it important to know which planet is closest to Earth?
It aids in planning space missions, enhances our understanding of the solar system, and enriches educational content in astronomy.